David Falk, 1932-2020
- Details
- Category: Department News
- Published: Friday, June 12 2020 15:32
Professor Emeritus David Sagal Falk died on June 10, 2020 at his home in Greenbelt.
Falk, a native of New York City, received his Ph.D. in 1959 at Harvard University. He held appointments at the University of Washington and as a NATO Postdoctoral Fellow at the Istituto di Fisica dell'Università, Genoa, Italy, before coming to the University of Maryland as part of an 11-person hiring spree directed by then-chair John S. Toll. At the time, Physics Today noted that the additions represented a 30 percent increase in the UMD physics faculty. Among those hired at the same time were Harry D. Holmgren, Guarang Yodh, Rolfe E. Gover, Arnold J. Glick, Richard E. Prange and Wally Greenberg.
Though his research was in condensed matter theory and statistical and thermal physics, his interests were broad. With Dieter Brill and David G. Stork, he wrote Seeing the Light: Optics in Nature, Photography, Color, Vision, and Holography, a very well-regarded and well-reviewed textbook published in 1986 and still popular today. He also created PHYS 106, the Physics of Light, which has taught thousands of non-majors about the wonders of holograms, lasers, sunsets, rainbows and other phenomena.
While serving as the physics department’s associate chair for education in the mid-1970s, he was instrumental in the establishment of the Slawsky Clinic, which has provided free tutoring to multitudinous UMD students. He also helped to create the department’s renowned lecture demonstration facility.
Falk was active on campus, serving on the Task Force on Academic Decision Making of the UMD Senate during a somewhat contentious 1970s overhaul of requirements in the campus curriculum and an enormous campus reorganization of departments and colleges that is still largely intact.
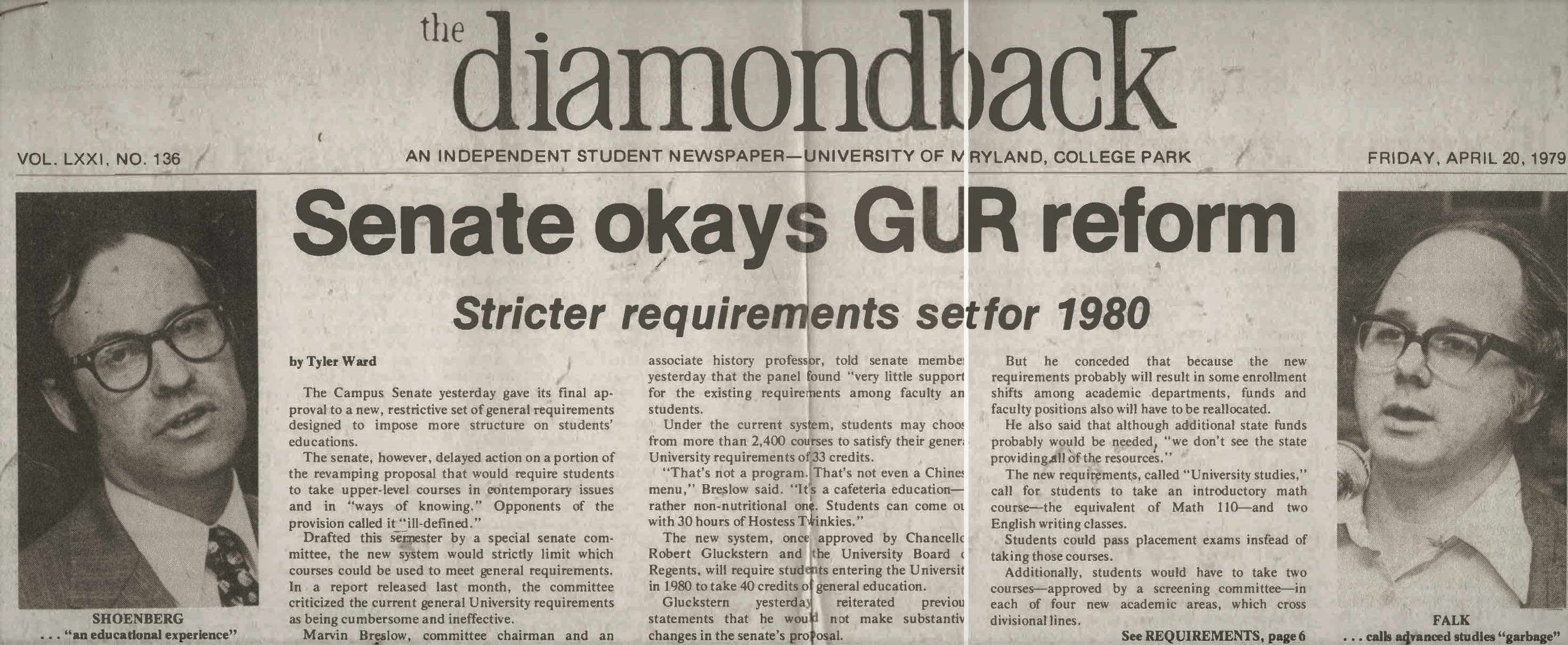
After Brit Kirwan was named Vice Chancellor for Academic Affairs in 1981, he enlisted Falk as Assistant Vice Provost. In this role, Falk oversaw curriculum development and managed academic interests in building construction and maintenance. He created a teaching facilities committee devoted to the upgrade of classrooms and the introduction of teaching technology. Falk continued in that position, working successively for Irv Goldstein, Bob Dorfman, and Jack Goldhaber until he retired in 1992 and was succeeded by another physicist, Victor Korenman.
Korenman recalls that Falk stressed quality control for this university. One example: during finals, Falk would walk the campus “to check that scheduled finals were actually being given, some faculty being inclined to give finals during the term, thus cutting down on actual instructional hours used.”
Falk also worked tirelessly to help guide the university during a 1990 financial crisis, and returned to campus in his retirement to assist with large projects such as the 1996 strategic plan.
Falk was known to be frank and plain spoken. He enjoyed funny hats, and when he left the Department of Physics for Academic Affairs, was bid adieu by the department with a "Mad Hatters" party.
The Falk family has asked that memorial trees be planted in his honor: https://www.legacy.com/obituaries/washingtonpost/obituary.aspx?n=david-falk&pid=196325612
The picture below shows the department around 1976. Falk is fourth from the left in the third row.
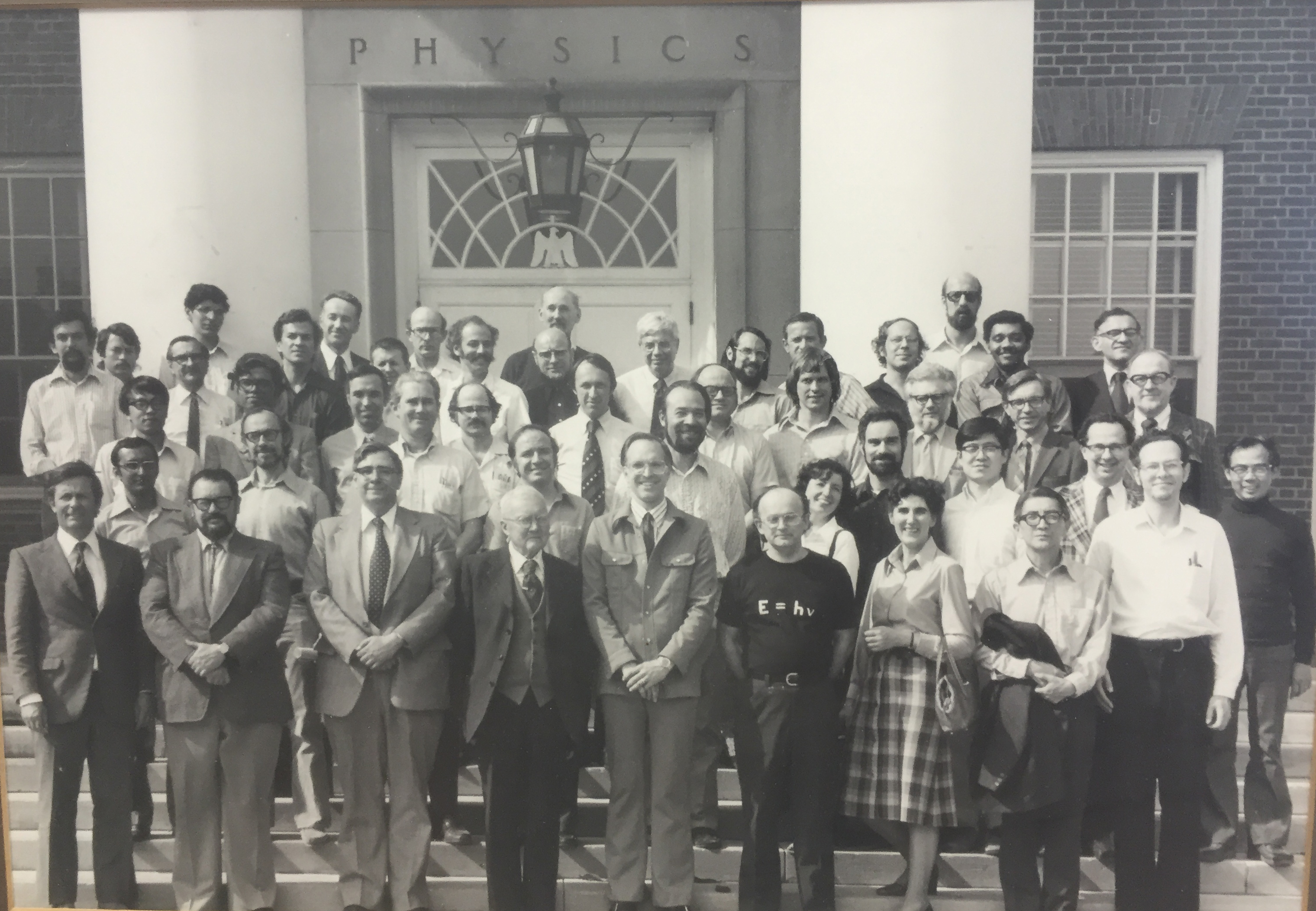